Ask the expert: Visiting ‘alternative Earths’ through the ocean’s past
Article Highlights
- Paleoceanographers are researchers who can examine ocean sediment like a record book that stretches back nearly 4 billion years.
- The evolution of photosynthesis has had major changes on ocean chemistry, but also the evolution of life in certain time periods.
- Phosphorus is thought to be one of the limiting nutrients for photosynthesis through a lot of Earth’s history.
- Ancient Earth can tell us about planetary conditions that sustain life that are very different from what we see today.
The Earth is 4.5 billion years old, and, during that time, it has seen some things. Life has been a part of most of that history, but what life has looked like has changed dramatically over the eons.
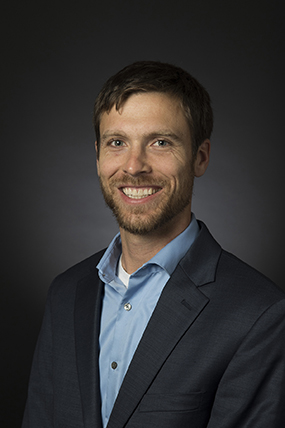
Dalton Hardisty, Geological Sciences Endowed Professor at Michigan State University. Credit: Michigan State University
Deciphering how life worked on this planet during its different epochs is one of the things that Dalton Hardisty works on at Michigan State University.
In fact, he’s part of an international research collaboration that recently published work in the prestigious journal Nature, sharing a new approach for studying important chemical and biochemical processes in the Earth’s prehistoric past.
He does this as part of a community of paleoceanographers, researchers who can examine ocean sediment like a record book that stretches back nearly 4 billion years.
MSUToday caught up with Hardisty, a Geological Sciences Endowed Professor in the Department of Earth and Environmental Sciences, to learn more about his field, why it’s important and what drew him to it.
What is paleoceanography?
Paleoceanography is the study of the evolution of Earth’s oceans through time.
Here at MSU, we focus on the biological and chemical evolution of the ocean. And it’s hard to distinguish between the two in lots of cases because they’re so intermingled. As life has evolved, it has changed the chemistry of the ocean in dramatic ways and vice versa.
A great example of that is oxygen in our atmosphere and how it has accumulated in the oceans. The evolution of photosynthesis has had major changes on ocean chemistry, but also the evolution of life in certain time periods.
What drew you to the field?
Paleoceanography is so cool. What drew me to it is this idea that we have these alternative Earths through time.
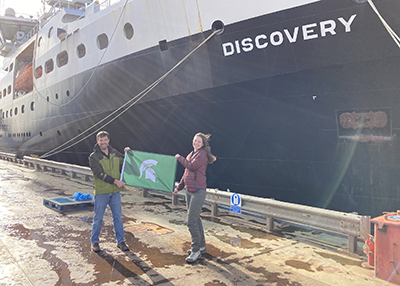
The Earth is 4.5 billion years old. The earliest records we have of ocean sediments go back to about 3.8 billion years, so we have 3.8 billion years of time to work with. That amount of time expands the number of interesting types of events that have occurred, but that we don’t see today.
There are mass extinction events. There are origination events. There’s the oxygenation of our atmosphere. It wasn’t until 2.5 billion years ago that we started to see clear signs of oxygen accumulation in our atmosphere, and it wasn’t until maybe somewhere in the last 540 million years that we see atmospheric oxygen become similar to today.
Across that entire time span, life has evolved dramatically and so it’s really exciting to be able to essentially get in your time machine and go back to learn about how the Earth works mechanistically, but also understand its history.
How can paleoceanography help us understand the planet today?
Things used to look very different from today, but they do matter for our present.
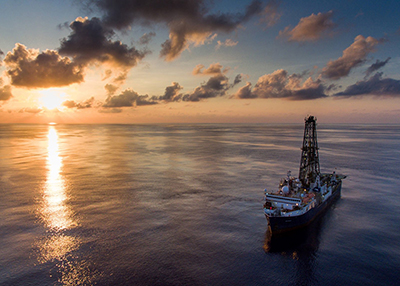
Right now, we can go out and observe and sample our oceans and atmosphere in amazing ways. But most observations of the chemistry of the oceans and the atmosphere don’t date back much beyond 100 years.
From there, we’re really relying on paleoceanography. That’s taking sediment records and reconstructing the changes in the chemistry of our oceans and atmosphere over natural warming events and natural increases in carbon dioxide. Then we can understand the baselines to expect and how to separate that noise out from what humans are driving currently.
It also lets us ask really basic questions about how life evolved. Why is Earth special? Is it special? We know that life exists here, and we’re curious about where it could exist elsewhere.
As we think about exoplanets and the search for life beyond Earth, the ancient Earth can tell us about planetary conditions that sustain life that are very different from what we see today.
What does the work of paleoceanography entail?
We look at the geochemistry of drill cores or from outcrops that you might find along the side of a road or a river or somewhere where there’s been erosion.
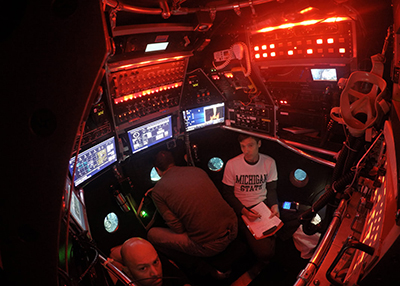
Dalton Hardisty, wearing a Michigan State T-shirt, sits with colleagues in a submersible vehicle during a voyage to hydrothermal vents to collect samples. Credit: Courtesy of Dalton Hardisty
But, also, we go out on ships and collect seawater samples and sediment cores to look at modern processes that give us some window into how we might understand this ancient record.
I love the field work. It is really a big part of paleoceanography. I’ve spent a couple of months working on a drill rig where we were sampling ocean sediments from the Baltic Sea. I’ve gotten to work in a submersible, where we went down and sampled directly from hydrothermal vents.
It’s exciting to be out there in real time. The ocean expeditions always humble you. You go out there and expect to see one thing, then you see very different things and have to figure out why that is the case.
Can you tell us a little bit more about the work that you were part of that was recently published in Nature?
The time period for this publication is around 600 million years ago, and this paper is interesting because there are two key periods, two key events that it looks at.
One is that there is a large change in Earth’s carbon budget. Across that same event, there is evidence for a diversification of some of the earliest animal life in the fossil record.
This paper also looks at oxygen and mechanisms that change oxygen, particularly phosphorus. We know that phosphorus is an important control on the availability of oxygen today, so we’re asking how important it was across some of these geologic events.
What makes phosphorus important?
Phosphorus is thought to be one of the limiting nutrients for photosynthesis through a lot of Earth’s history.
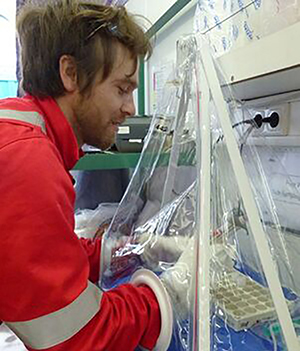
Dalton Hardisty of Michigan State University works with water samples found in sediment during a research expedition on the Baltic Sea. Credit: Courtesy of Dalton Hardisty
We see this even today with nutrients from fertilizer for our gardens like nitrogen, phosphorus and potassium that can run off into lakes, rivers and oceans. Those nutrients and their availability have long limited the growth of primary producers, organisms that do photosynthesis like algae and cyanobacteria.
For example, in Lake Erie, there are large inputs of phosphorus and, every summer, there are these large algal blooms that change the oxygen concentration in the lake. These are big hypoxic events where a lot of oxygen is lost in the water column and that’s linked to phosphorus.
What were the results of your paper?
What we published is a new geochemical approach in which we looked at phosphorus across those key events in the past. That let us develop a model to look at the impacts of phosphorus on oxygenation, play with it and ask, “Okay, can we actually mimic what we see?”
So there will be a need for some validation that this approach is working across other events. But it’s a way to mechanistically understand how phosphorus has changed through Earth’s history and how it has — or has not — impacted oxygen accumulation and photosynthesis broadly.
What makes MSU a good place to do this type of research?
I’ve had several people ask me why I study the ocean here when MSU isn’t adjacent to a coast. And, while it’s true a lot of oceanography programs are on coastlines, I don’t think it matters at all. People who study Antarctic ice sheets don’t need to live in Antarctica, for example.
What MSU offers is a really amazing laboratory that we’ve built up for the types of analyses we want to do. The other thing is that, by its nature, this type of work is interdisciplinary, and our department is very interdisciplinary.
That’s what I love about the earth sciences. We have geomicrobiologists, we have geophysicists, and we have other people who study the ocean from different perspectives. So, we can collaborate on some of these important problems as we try to understand the evolution of life and the Earth.Banner: Scientific drill ships, such as the JOIDES Resolution seen here, extract samples from the ocean floor that help researchers like Dalton Hardisty of Michigan State University reveal Earth’s prehistoric past. Credit: Shuhao Xie/International Ocean Discovery Program and JOIDES Resolution Science Operator