Wired in: Simulating how electrons move through nanowires
Article Highlights
- Michigan State University researchers are studying biological nanowires—microscopic wires made of proteins—that have caught attention for their ability to carry electrons over long distances.
- The team created simulations of crystals, where they pointed a light source at a nanocrystal made up of proteins and calculated how fast excited electrons traveled through it.
- When variables other than temperature were manipulated, the researchers started to see some interesting action from the electrons’ hops within the nanowire.
- Understanding how biological nanowires can be constructed to allow for more electron flow is crucial to future endeavors using them to connect biological processes to conventional electronics.
The movement of electrons across wires is what allows us to use electricity every day. Biological nanowires—microscopic wires made of proteins—have caught researchers’ attention for their ability to carry electrons over long distances.
In a recent study published in the journal Small by the Josh Vermaas lab in the Michigan State University-Department of Energy (MSU-DOE) Plant Research Laboratory (PRL), researchers expand our understanding of biological nanowires through the use of computer simulations.
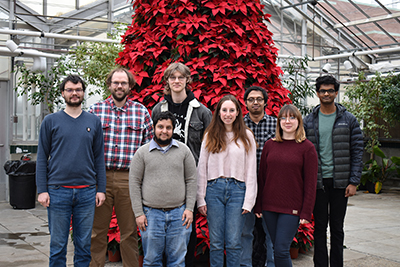
Martin Kulke, a postdoc in the Vermaas lab and a first author of the study, worked with the team to create simulations of crystals using data from the real-life experiments in the PRL’s David Kramer lab, where they pointed a light source at a nanocrystal made up of proteins and calculated how fast excited electrons traveled through it. The real question was why electron transfer was getting slower with increasing temperature, which usually accelerates processes at the nanoscale.
One potential idea was that the distances electrons would need to jump within the nanocrystal might increase with temperature, slowing down how fast they could move through the protein.
“We simulated these protein nanocrystals at different temperatures to test this idea,” said Vermaas, assistant professor in the Department of Biochemistry and Molecular Biology in the College of Natural Science, PRL faculty member and the study’s primary investigator. “What we found is that the distance changes across different temperatures are not so dramatic on their own.”
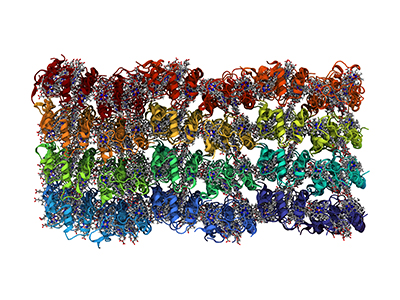
In this representation, each of the 96 proteins in the nanocrystal are a different color. The electrons travel from heme group to heme group inside the protein. The hemes are shown in a stick representation, with gray for the carbons, blue for the nitrogens and a pink iron atom. Credit: Vermaas lab
When variables other than temperature were manipulated, the researchers started to see some interesting action from the electrons’ hops within the nanowire. The nanowire protein network was made longer, shorter, thicker and thinner to identify bottlenecks to the electron flow within the nanocrystal.
“We found that in biological nanowires, the electron transport is based on the motion of the proteins in the wire,” Kulke said. “What that means is in the end, the longer you make those nanowires, the less electron transport you get through them and the thicker you make them, the more electron transport you get through them.”
The use of biological nanowires is speculative at the moment, but understanding how they can be constructed to allow for more electron flow is crucial to future endeavors using them to connect biological processes to conventional electronics.
This research was supported in part by the U.S. Department of Energy, Office of Basic Energy Sciences, the XSEDE EMPOWER program under the National Science Foundation and computational resources and services provided by the Institute for Cyber-Enabled Research at Michigan State University.Banner image: A rendering of a protein nanowire (yellow) cutting through a protein blob (gray) with electron carriers (orange) traveling along it. Credit: Martin Kulke