MSU helps make cosmic discovery
For just the second time in human history, researchers have identified a source of high-energy neutrinos — ghostly subatomic particles produced in some of the universe’s most extreme environments.
The discovery was made by an international collaboration led by Michigan State University and Technical University of Munich researchers at the IceCube Neutrino Observatory in Antarctica. The team announced its findings on Nov. 3 in an online webinar and will publish its study Nov. 4 in the journal Science.
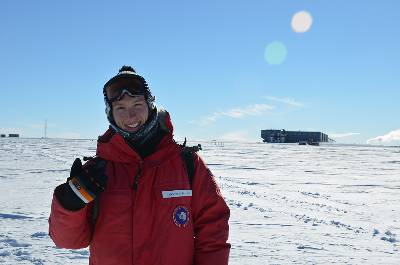
MSU postdoctoral research associate Hans Niederhausen in Antarctica. Credit: Sam De Ridder, IceCube/NSF
The first high-energy neutrino source was discovered in 2018 and was also made possible by IceCube. In both cases, scientists found neutrinos were coming from galaxies with central black holes actively gobbling up matter. But the sources also differ in important ways.
For one, the newly discovered source could prove to be more common than what was discovered in 2018. Also, in the case of the new source, its neutrinos carry information about their origin that’s inaccessible to other types of telescopes, including those that detect high-energy gamma rays.
This helps advance an idea that’s been around since the 1960s, that with the technology and techniques to study cosmic neutrinos, we can open new windows of exploration and understanding into our universe.
“We’re not all the way there yet,” said Hans Niederhausen, a lead author of the new report and postdoctoral research associate in the Department of Physics and Astronomy in the MSU College of Natural Science. “But this could be the point we look back to and say, ‘This is when we showed we can do it in practice.’”
The truth is out there
In the early 20th century, scientists discovered that extremely energetic particles from the cosmos were colliding with Earth. These were cosmic rays, not neutrinos, but physicists believe the two types of particles are made in the same cosmic cauldrons.
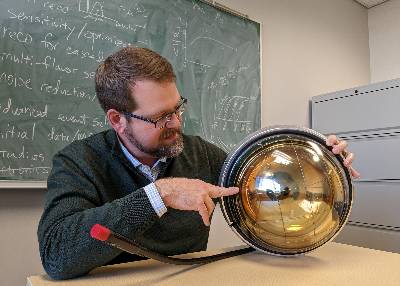
MSU Professor Tyce DeYoung and a digital optical module, just like the ones embedded in Antarctica’s ice at IceCube used to detect neutrinos. Credit: Matt Davenport/Michigan State University
After 100-plus years of directly detecting cosmic rays, though, scientists still don’t know exactly what the most energetic sources of these particles are or what’s going on inside them.
For context, these sources produce particles that have more energy than those ejected by a supernova, which is an exploding star. These high-energy particles carry millions of times more energy than what we’re able to create on Earth with our most powerful particle accelerator.
“These are conditions that we will never, ever replicate on Earth,” said Tyce DeYoung, a professor in Department of Physics and Astronomy and a leader of MSU’s IceCube research contingent.
Being able to study high-energy neutrinos would create new ways to probe questions about the environments that produce them.
“We’ve known for over a century that there are cosmic accelerators producing particles with ridiculously high energy. For more than 100 years now, they’ve represented something that we know we don’t know about the universe,” DeYoung said. “It’d be nice to finally have some answers.”
Here’s the rub. Neutrinos are notoriously difficult to detect. They have no electric charge and almost no mass. It’s exceedingly rare for them to interact with normal matter.
In fact, trillions of neutrinos fly through you every second without so much as a “hello.” That’s why they’ve earned the nickname “ghost particles.”
The idea of using a new type of telescope to detect cosmic high-energy neutrinos has been around since the 1960s. But it wasn’t until 2013 that scientists finally saw these energetic ghost particles from the heavens.
That discovery — named Physics World magazine’s Breakthrough of the Year — was made by IceCube, a collaboration that includes 58 institutions spanning 14 countries with support from the National Science Foundation and other agencies.
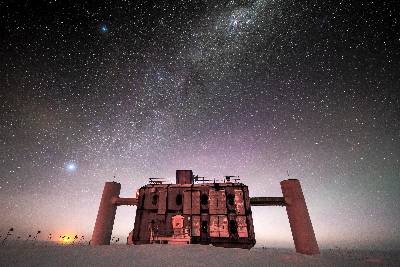
IceCube at twilight, with a starry sky showing a glimpse of the Milky Way overhead and sunlight lingering on the horizon. Credit: Martin Wolf, IceCube/NSF
“Answering these far-reaching questions about the universe that we live in is a primary focus of the National Science Foundation,” said Denise Caldwell, director of NSF’s Physics Division.
Researchers can’t make neutrinos interact more with matter, but they can give neutrinos more matter to potentially interact with. It’s similar to how every lottery ticket has the same small odds of being a winner, but buying more tickets improves someone’s odds of winning.
At IceCube, the matter comes in the form of a cubic kilometer of Antarctic ice, a billion tons of frozen water. Within the ice, researchers have embedded more than 5,000 modules that look for signs of neutrinos.
The observatory detects tens of thousands of neutrinos per year, but only a few hundred per year are from cosmic sources. Of those, only a few dozen have energies high enough to be identified individually as cosmic in origin. That’s not a lot, but it’s been enough for IceCube.
A tale of two galaxies
Since 2013, whenever IceCube researchers would detect a high-energy cosmic neutrino, they’d first triangulate where in the universe it came from. They’d then reach out to their astronomer colleagues and ask if anything interesting was happening in that celestial neighborhood, DeYoung said.
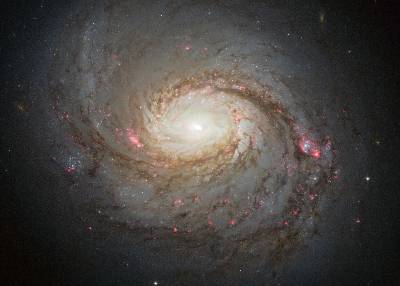
MSU researchers have helped show that the galaxy NGC 1068, also known as Messier 77, is a cosmic neutrino source, making it the second known high-energy neutrino source in our universe. Credit: NASA/ESA/A. van der Hoeven
The answer was yes for one neutrino that hit IceCube in September 2017. The supermassive black hole at the core of an active galaxy called TXS 0506+056 was hurtling high-energy particles toward Earth. In 2018, this active galaxy, which is a type known as a blazar, was confirmed as the first-known source of high-energy neutrinos.
“When we found that source in 2017, we started saying, ‘We know there are other things like this in the universe. Are they producing neutrinos?’” DeYoung said.
Members of the particle astrophysics community put their heads together to come up with an estimate of how much of the universe’s high-energy neutrino budget could be accounted for by TXS 0506+056 and its ilk. That number was about 8% at most, DeYoung said.
Put another way, it’s probably not a typical high-energy neutrino source, which was a little unexpected. Things like blazars are exactly what many had suspected would be producing lots of high-energy neutrinos because they were producing lots of other high-energy particles scientists were already observing.
“We had our list of usual suspects, and we were looking for the things we already knew were part of the high-energy universe,” DeYoung said. “It started looking like maybe we weren’t chasing down the right path.”
The new discovery gives credence to that assessment. The newly confirmed source is a galaxy called NGC 1068, also known as Messier 77. It also has an active black hole at its core, but its high-energy cosmic rays are shrouded in a cloud of dust.
“If you’re looking for its high-energy gamma rays, it’s not exciting,” DeYoung said. “In terms of photons, it looks boring at high energy.”
“We’re seeing its neutrinos much more strongly than its gamma rays,” Niederhausen said. “That helps us understand the object beyond just saying there are neutrinos associated with it.”
“I think NGC 1068 could become a standard candle for future neutrino telescopes,” said Theo Glauch, another lead author of the study and a postdoctoral associate at the Technical University of Munich, or TUM, in Germany. “Neutrinos will allow us to see this galaxy in a totally different way. A new view will certainly bring new insights.”
“The unveiling of the obscure universe has just started, and neutrinos are set to lead a new era of discovery in astronomy,” said Elisa Resconi, a professor of physics and head of the TUM IceCube group.
Ghost (particle) hunters
Researchers had suspected NGC 1068 to be a neutrino source for decades, but proving it required refining IceCube’s data and analysis to pinpoint it with the necessary accuracy.
Niederhausen and Glauch were instrumental in this, developing new statistical and machine-learning methods to search for neutrino sources. Still, Niederhausen stressed this was made possible by building on the past and ongoing efforts of the larger collaboration.
“We were able to zoom in and make the analysis more precise, but the story doesn’t rest only on that. There are levels of work that made this possible across all of IceCube,” Niederhausen said.
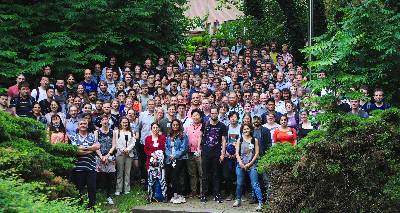
IceCube Collaboration members attending the first in-person meeting after two years of virtual meetings, in Brussels, Belgium spring 2022. Credit: IceCube Collaboration
“Work by the detector operations and calibrations teams enabled better neutrino directional reconstructions to precisely pinpoint NGC 1068 and enable this observation,” said Ignacio Taboada, a physics professor at the Georgia Institute of Technology and the spokesperson of the IceCube Collaboration.
“Resolving this source was made possible through enhanced techniques and refined calibrations, an outcome of the IceCube Collaboration’s hard work,” he said.
It’s also noteworthy that NGC 1068 is among the best-studied active galaxies in astronomy. Unlike the case with the first confirmed high-energy neutrino source, IceCube didn’t need to work directly with astronomers to discover what was happening where the neutrinos were coming from. That was already well-documented.
NGC 1068 is also what’s known as a Seyfert galaxy and is inherently different from the blazar source discovered earlier. Remember, that blazar and similar sources are producing at most 8% of the universe’s high-energy cosmic neutrinos.
It’s still too early to know whether sources like NGC 1068 will prove to be more common, but this new report confirms they will account for at least some portion of that outstanding 92%.
As researchers work on that piece of the puzzle, others will focus on what the neutrinos from sources like NGC 1068 tell us about active galactic cores that we can’t learn from other particles.
“IceCube has accumulated some 80 neutrinos of teraelectronvolt energy from NGC 1068, which are not yet enough to answer all our questions,” said Francis Halzen, a professor of physics at the University of Wisconsin–Madison and principal investigator of IceCube. “But they definitely are the next big step toward the realization of neutrino astronomy.”
The IceCube Neutrino Observatory is funded and operated primarily through an award from the National Science Foundation to the University of Wisconsin–Madison (OPP-2042807 and PHY-1913607). The IceCube Collaboration, with about 350 scientists in 58 institutions from around the world, runs an extensive scientific program that has established the foundations of neutrino astronomy. https://icecube.wisc.edu/collaboration/institutions
IceCube’s research efforts, including critical contributions to the detector operation, are funded by agencies in Australia, Belgium, Canada, Denmark, Germany, Italy, Japan, New Zealand, Republic of Korea, Sweden, Switzerland, Taiwan, the United Kingdom, and the United States. The IceCube EPSCoR Initiative (IEI) receives additional support through NSF-EPSCoR-2019597. IceCube construction was also funded with significant contributions from the National Fund for Scientific Research (FNRS & FWO) in Belgium;
the Federal Ministry of Education and Research (BMBF) and the German Research Foundation
(DFG) in Germany; the Knut and Alice Wallenberg Foundation, the Swedish Polar Research
Secretariat and the Swedish Research Council in Sweden; and the University of Wisconsin–Madison
Research Fund in the U.S.
Banner Image: As part of the IceCube Collaboration, Michigan State researchers are using neutrinos to probe the inner depths of an active galaxy. Credit: NASA/ESA/A. van der Hoeven